Incorporating growth factors into scaffolds to reduce costs and introduce spatial heterogeneity
Growth factors (GFs) can be incorporated into scaffolds as a strategy for both reducing costs and improving product quality of cultivated meat. Open-access research is needed to test the feasibility of this strategy and determine the most appropriate methods.
-
Cultivated
- Research
- Commercial
- End Products
- Production
- R&D
- Scaffolding
- Industry
- Academics
- Startups
Current challenge
Today, the cost of growth factors (GFs) and other recombinant proteins is a substantial contributor to the cost of producing cultivated meat. However, their contribution to the total production cost is likely to decrease—both in absolute terms and as a fraction of the total cost—with increased scale and improved technology. These costs can be estimated per kilogram of meat, liter of culture media, or milligram of GF based on current usage levels of GFs in cell culture media formulations. However, the former two categories likely assume a higher-than-necessary usage level of GFs because the vast majority of the GF molecules in the process are floating freely in the media rather than in direct contact with the target cells.
GFs and other proteins per kg meat
In the scenario modeled by Humbird in 2021, GFs only contributed 8—11% of the total production cost of cultivated meat. However, this still accounts for USD $3—4/kg meat and therefore would have to be further reduced for cultivated meat to become competitive with commodity meat prices of USD $2/kg, even if all other costs were reduced to near-zero. In the baseline low media use scenario modeled by Vergeer et al. in 2021, GFs (TGF-β and FGF2) added USD $33/kg, or 22%, to the total production costs, while other recombinant proteins (insulin, albumin, and transferrin) contributed USD $99/kg or 66%. These authors estimated that cost reductions of 1000-fold and 500-fold might be feasible. With these reductions, the total contribution of GFs and recombinant proteins would be a tolerable USD $0.23/kg.
GFs and other proteins per liter media
GFI’s 2018 analysis of Essential 8 media estimated that 99% of the cost in the baseline scenario (USD $373/L) came from FGF2, TGF-β, insulin, and transferrin (primarily FGF2 and TGF-β). This was reduced to USD $0.12/L (primarily insulin and transferrin) in the most optimistic scenarios for cost reduction of GFs and recombinant proteins, which accounted for 3—50% of the total media cost.
Subsequent reports based on researchers’ attempts to reduce the cost of serum-free media have validated the assumption that cost reduction is feasible, though more progress is needed. Kuo et al.* in 2020 reported a combined cost for GFs and recombinant proteins in B8 media of USD $2.75/L, or about 25% of the total media cost, when produced at lab scale. Using commercial suppliers in 2021, Stout et al.* reported an estimated contribution of GFs of USD $5—30/L Beefy-9 media and other recombinant proteins of ~USD $40-100/L media. In all scenarios, the total contribution of recombinant proteins and GFs was more than 50% of the total media cost. In 2022, Venkatesan et al. reported producing TGF-β and FGF2 in-house for USD $0.82**/L media (4% of total media cost, compared to 95% when buying from commercial suppliers). Assuming the use of 20,000 L media per batch (“low media use”) and a yield of 3,500 kg meat per batch as in GFI’s Essential 8 analysis mentioned above, a cost contribution of USD $0.82—30/L of media corresponds to USD $4.69—171.43/kg.
Cost per gram of GF or other proteins
While the price of some commercially-available GFs has come down dramatically in recent years, in part due to pressure for sources suited to the cultivated meat industry, cost remains a challenge. ORF Genetics offers bovine, porcine, and avian FGF2 human FGF2 for USD $196** per mg (as of this writing in September 2022), compared to the USD $7.47** per mg reported by Venkatesan et al. For comparison, the price modeled in the 2018 analysis was the equivalent of USD $2,005 per mg.
Achieving complexity in cultivated meat
Reducing the production costs of cultivated meat means little if the quality and variety of the available products do not match or exceed that of conventional meat. Skeletal muscle is a complex tissue made up of myofibers, adipocytes, and smaller numbers of other cell types. The biological and mechanical properties of muscle tissue ultimately determine the organoleptic properties of meat. Cultivated meat may not need to replicate all of this complexity. However, it is a safe assumption that producing whole-cut products that are the same or better than conventional meat from a flavor and texture perspective will be a substantial challenge. In particular, certain types or cuts of meat where a heterogeneous distribution of cell types is key to the meat-eating experience—such as marbled steaks or fish filets from fattier species—will need to account for this complexity. It may be difficult to achieve a heterogeneous product with multiple cell types responding to different differentiation cues without some spatial control over the signals these cells are receiving. When growth factors are free-floating in the cell culture media, it is not possible to co-culture different cell types without the risk of them responding to all of the signals in the media.
*Costs mentioned here based on the work of Kuo et al. and Stout et al. were estimated from the paper text and figures and might not exactly match equivalent estimates by others based on the same papers.
**Converted to USD from CAD or € (March 15, 2022)
Proposed solution
Incorporating GFs into scaffolds or microcarriers could improve the cost, scalability, and organoleptic properties of cultivated meat in several ways:
- This strategy could reduce the total amount of GF required to achieve the desired effect on cell proliferation or differentiation.
- Achieving a slow, sustained release exactly where the GF is needed could reduce the need to either recycle GFs from the media or add GFs throughout the culture period, thereby simplifying the bioprocess.
- GFs could be incorporated in a heterogeneous pattern, providing additional spatial cues to guide cell attachment or differentiation in complex, whole-cut cultivated meat. Similarly, tuning the release kinetics of multiple GFs could facilitate the transition from proliferation to differentiation within the same scaffold.
Sustained release of encapsulated GFs has been reported in multiple studies. For example, RADA16 self-assembling scaffolds with or without incorporated TGF-β were used to grow bone-derived mesenchymal stem cells. In this study, TGF-β was gradually released over a period of at least 7.5 days. Similarly, BSA or PDGF encapsulated within chitosan microspheres was released at an even rate from day 1 through day 28 of incubation in phosphate-buffered saline, though the kinetics may differ somewhat in the presence of media and cells. Encapsulation of insulin and transferrin within scaffold materials has also been demonstrated.
While the strategy of simply encapsulating GFs within a scaffold has shown promise, for some GFs or applications, it may be desirable to further constrain diffusion. Compared to native bFGF incorporated into the same collagen-based scaffold, bFGF fused to a collagen-binding domain was found at higher levels between 1 and 7 days after in vivo implantation and was more effective at supporting nerve regeneration. This method could be applied to scaffolds derived from other materials. For example, cellulose-binding domains could anchor GFs onto plant-derived scaffolds (see Related Efforts for more examples of immobilization strategies).
In addition to constraining GF diffusion from scaffolds, there is also precedent for incorporating GFs into scaffolds in a heterogeneous pattern. For example, scaffolds were produced from electrospun fibroin containing a concentration gradient of NGF across the scaffold length. For cultivated meat, spatially heterogeneous GF patterning could help mimic the natural marbling or other patterns found in conventional meat, thereby encouraging the growth of muscle, fat, and other tissues in defined regions.
Furthermore, the effective concentration of multiple GFs in a scaffold could be varied in a time-dependent manner by relying on different release kinetics. This “temporal heterogeneity” could be used to promote proliferation when the cells are initially seeded onto the scaffold, followed by differentiation and maturation as different GFs become available over time.
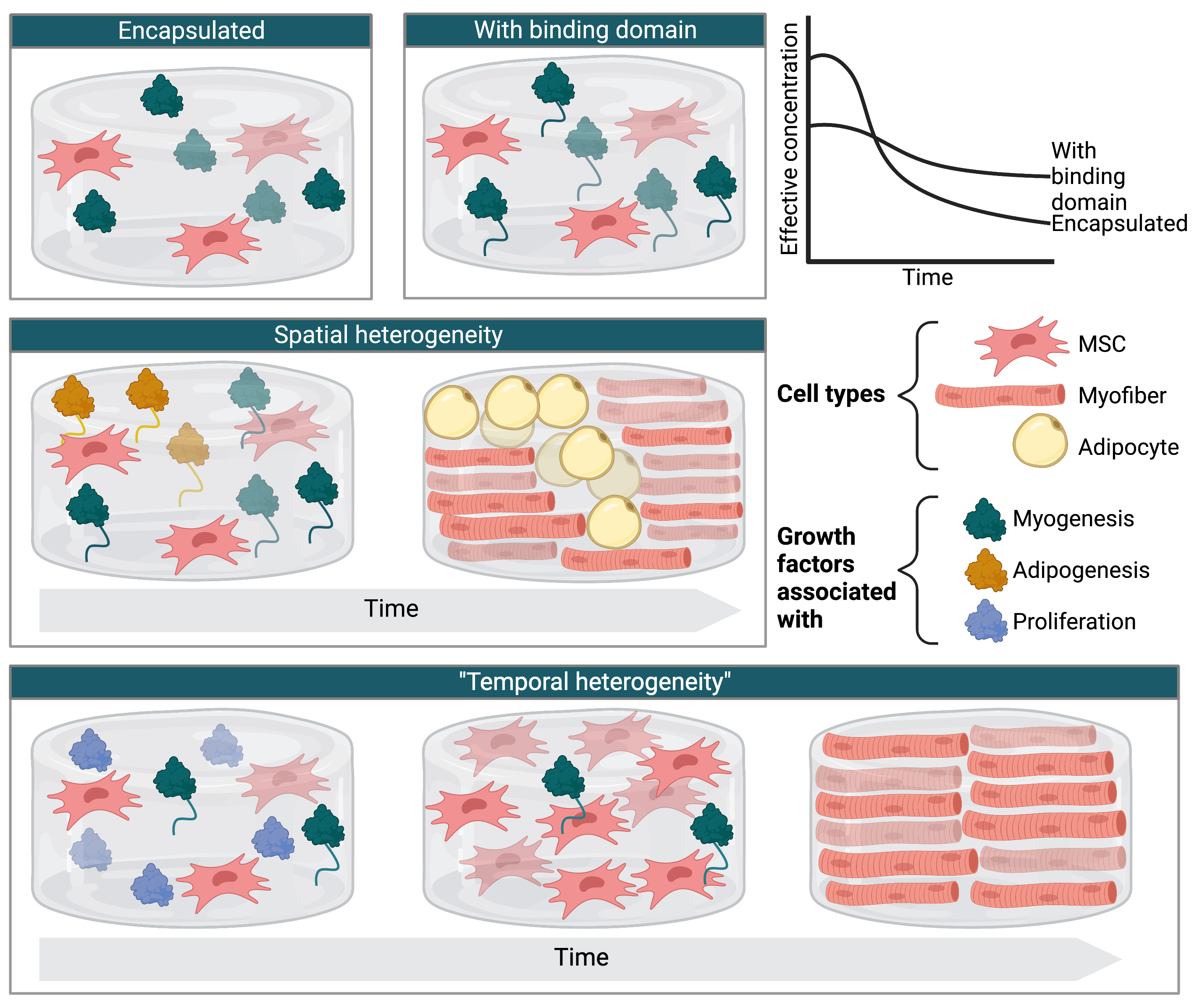
GFs could be incorporated into scaffolds in several possible ways. Top row: GFs may be simply encapsulated or anchored more strongly using binding domains, which has been demonstrated to further slow GF release. Middle row: Scaffolds with spatially heterogeneous GF distributions could be used to achieve marbling or other desired patterns via differentiation of multipotent cells such as mesenchymal stem cells (MSCs). Bottom row: Multiple GFs could be incorporated with different release kinetics to support proliferation and differentiation on the same scaffold. Created with Biorender.com.
Anticipated impact
One likely benefit of constraining GFs within scaffolds or microcarriers is a reduction in the total amount of GFs needed to induce a given cellular response and, therefore, the cost of producing a cultivated meat product. There may also be advantages from a regulatory and consumer perception perspective if the total amount of GF used in the production process can be reduced to amounts similar to those present in naturally occurring animal muscle tissue.
Several other approaches have the potential to achieve similar reductions in the contribution of GFs to the cost of cultivated meat. These include improving methods for producing recombinant GFs, taking advantage of economies of scale, and developing systems for media recycling. Multiple approaches may allow flexibility for cultivated meat companies to optimize their production systems and lead to more successful cost reductions with less time and effort by taking advantage of the “low hanging fruit” of multiple strategies. For example, it may be easier to reduce costs by ten-fold each with two methods than to achieve a hundred-fold reduction with one.
One helpful output from early tests of GF incorporation into scaffolds would be estimates of the increase in the “effective” GF concentration achievable. That is, by how much can the total amount of GF be reduced while maintaining the desired cellular response? While further optimization will achieve better results, open-access research making these estimates can indicate the order of magnitude of benefit that might be achievable. This will either increase the field’s confidence in this approach or allow it to be discarded in favor of alternative routes before more time and money is spent.
Tuning the concentrations of GFs—or other molecules—in space and time may further the ability of the cultivated meat industry to deliver on its promise of providing a variety of meat cuts that are competitive with their animal-derived counterparts. This strategy may be especially important for complex cuts of meat, including those where bone, cartilage, or other tissues play an important role.
Related efforts
In an anonymous survey conducted by GFI and TurtleTree Scientific in 2020, while the majority of companies surveyed indicated that they plan to use soluble growth factors, several companies had plans to use scaffolds or microcarriers with tethered or microencapsulated GFs.
Methods for immobilizing GFs or other reagents on scaffold materials include:
- Carbodiimide crosslinking (e.g. Odedra et al. 2011, Madl et al. 2014, Olde Damink et al. 1996)
- Biotin-avidin (e.g., Hu et al. 2013)
- Click chemistries (e.g., Hudalla et al. 2010)
- Collagen binding domains (e.g., Ma et al. 2014)
- Immobilized heparin (e.g., Moore et al. 2018)
- Layer by layer self-assembled BMP-2 and VEGF (e.g., Macdonald et al. 2011)
- Polydopamine-based bioconjugation (e.g. Cho et al. 2014)
Banks et al. used carbodiimide crosslinking and benzophenone photoimmobilization to orthogonally control scaffold stiffness and concentration of platelet-derived growth factor (PDGF) or bone morphogenetic protein (BMP)-2.
Mvula and Abrahamse co-cultured adipose-derived stem cells and smooth muscle cells in 2D conditions with or without GFs, and observed decreased expression of adipose markers and a non-significant increase in expression of myosin heavy chain in response to TGF-β treatment.
A review by Abitbol et al. on applications of nanocellulose discusses cellulose binding domains as a promising approach for the development of nanocellulose-protein composite materials.
A review by Wang et al. discusses the use of controlled growth factor release in scaffolds developed for biomedical application.
Park et al. (2021a) described a method for the controlled release of C-phycocyanin from chitosan/carboxymethylcellulose sheets capped with agarose for use in serum-reduced cultivated meat.
Park et al. (2021b) used gelatin microspheres for controlled release of C-phycocyanin and IGF-1 to support the growth of cultivated meat prototypes.
GFI resources
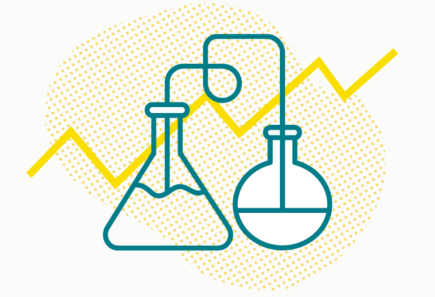
Cultivated meat media and growth factor trends
GFI and TurtleTree Scientific have partnered to distill a snapshot of current cultivated meat industry progress and needs, with an eye toward future demands and cost reduction prospects, based on…
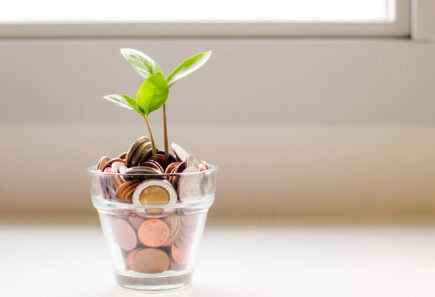
Research funding database
GFI’s research funding database provides curated grant opportunities for open-access alternative protein research.
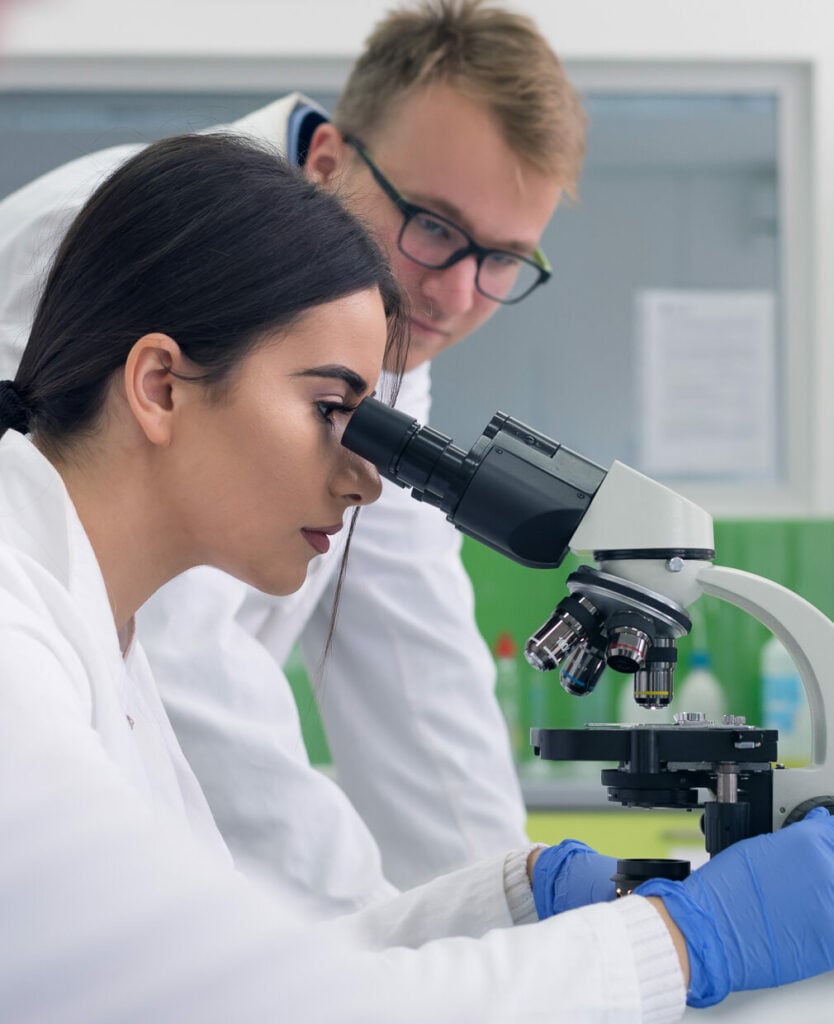
Find collaborators
Join the GFIdeas global community of 2,000+ entrepreneurs, scientists, investors, and subject matter experts. Discuss projects on the members-only Slack community, attend monthly seminars, and use the community directory to help you find collaborators working on similar Solutions!
Related solutions
-
Cultivated
-
Fermentation
Systematic investigation of growth factor needs and effects
Open-access research into growth factors required for proliferation, maintenance, and differentiation of cell types relevant to cultivated meat will support both academic and industry research efforts. This research could include…
-
Cultivated
B2B growth factors for proliferation
There is a need for a supplier of low-cost growth factors produced without the use of animals to support the proliferation phase of cultivated meat production. The cost of growth…
Related GFI research grants
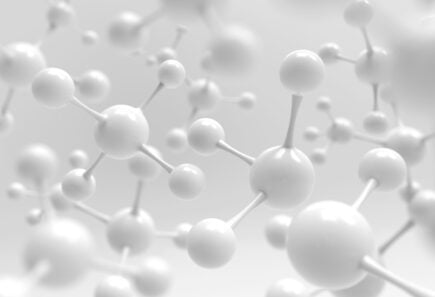
Formulating media with macromolecular crowding
Learn about Dr. Connon and Dr. Gouveia’s work at Newcastle University, UK to formulate growth media for cultivated meat with macromolecular crowding.
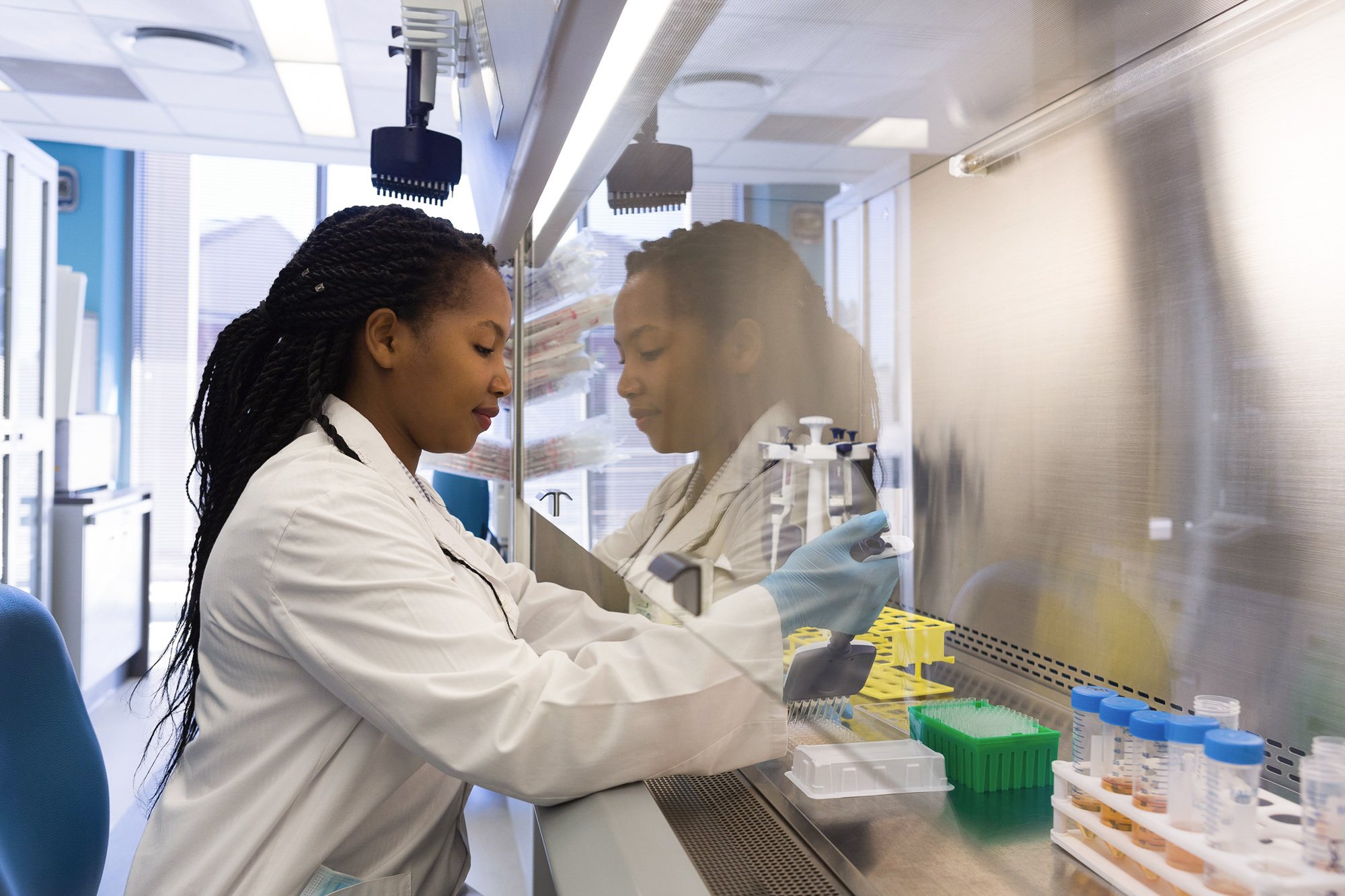
Explore the full solutions database
Browse 100+ startup ideas, commercial opportunities, research projects, and investment priorities throughout the alternative protein supply chain.
Get involved
If you’d like to fund a research project, work on any of these solutions, share information about related efforts that are already underway, or elevate new ideas for advancing the alternative protein industry, we’d love to hear from you!