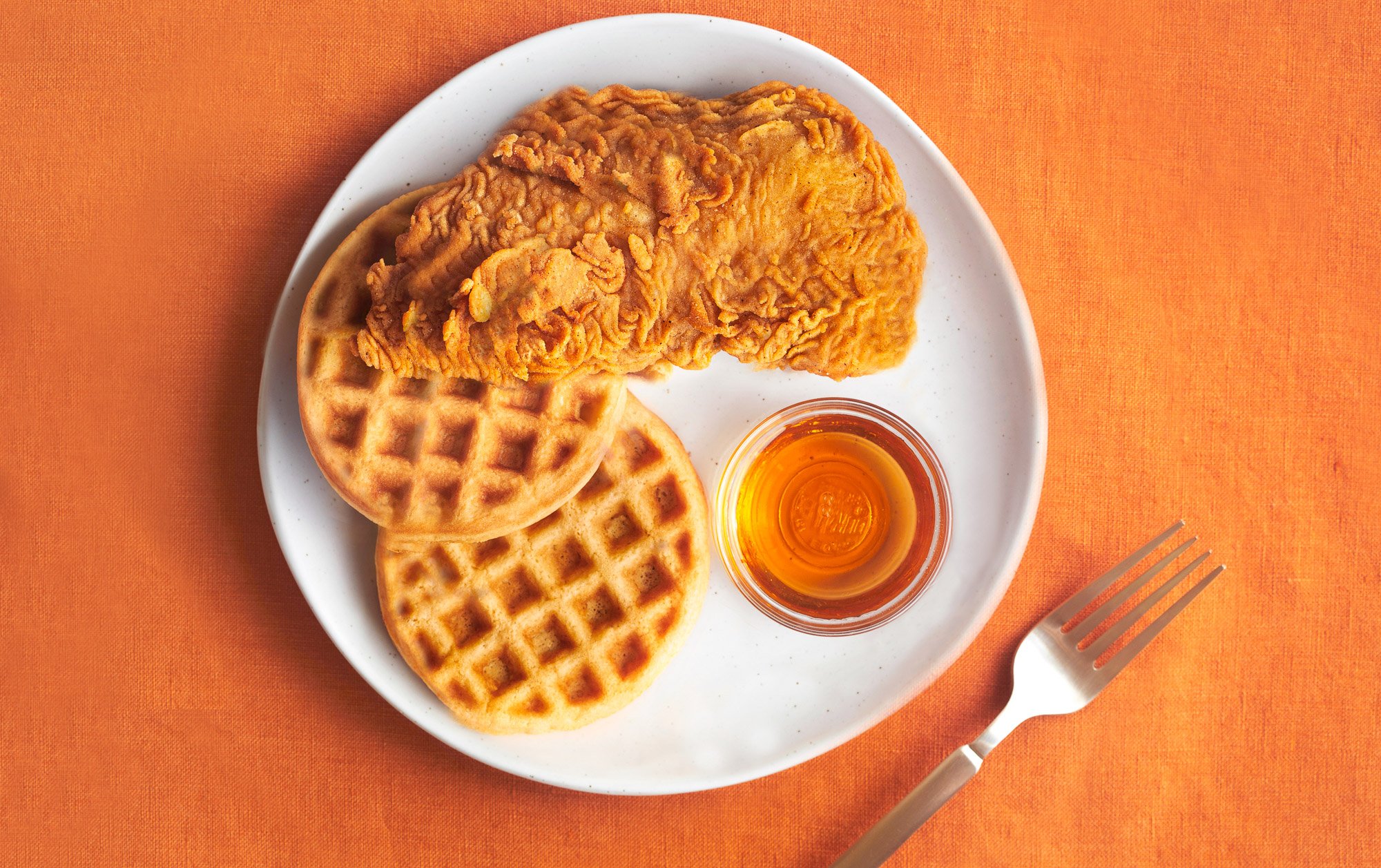
Preliminary review of technical assumptions within the Humbird analysis
GFI scientists expand on the limitations of single studies for assessing the full range of technological possibilities
Overview
Overall, the Humbird report presents a thoroughly referenced, detailed analysis of the current challenges of pursuing scale-up of cultivated meat in fed-batch and perfusion-based stirred-tank reactor systems, which by themselves represent methods that cultivated meat manufacturers are currently pursuing but do not necessarily represent the full range of scale-up (e.g., here) or biomass-generating methodologies (e.g., here and here). This analysis is valuable for identifying and prioritizing areas where additional innovation is needed to overcome the current limitations. However, this analysis incorporates several assumptions and interpretations and that we feel merit further discussion or fail to acknowledge the current state of the science (e.g., where researchers or companies are already developing promising technologies that address said issue). We have enumerated several of these points below, in the hopes of providing clarity on some of the nuanced reasons that different TEAs reach different conclusions at this speculative stage of development of this emerging technology.
100 kTA global production is likely not beyond the realm of novelty pricing.
The assumption that “any extravagant price premiums associated with product novelty have expired” at the 100 kTA scale (the approximate scale of plant-based meat today), and that this reflects “a waypoint in the development of adoption of cultured meat, one that should be squarely beyond the ‘valley of death’ associated with new bioproduct development” merits reassessment. Beyond Meat and Impossible Foods still charge price premiums at their current scale, and their explicit goal is to be as mainstream and accessible as possible, as quickly as possible. In fact, many markets are clamoring for these products and would pay higher price points than here in the U.S. (as evidenced by select international markets that have succeeded in striking deals), but these companies have mostly chosen instead to expand their base at home rather than export at top dollar.
According to Our World in Data, global terrestrial meat production in 2018 totaled 341,160 kTA, and global seafood production in 2013 totaled 154,850 kTA. According to Beef Magazine, specialty products (in this case defined as grass-fed beef and pasture-raised chicken and pork) account for 5% of beef sales and 1% of chicken and pork sales volume in the United States. If we extrapolate this to worldwide sales of all meat and seafood, taking the more conservative 1% as the cutoff for “specialty” products, we would estimate a global production volume of 6,000 kTA of high-end meat and seafood. 100 kTA of cultivated meat and seafood represents only 0.017% of total production, or 1.7% of the high-end market. It therefore seems entirely possible that cultivated meat could replace 100 kTA globally of strictly high-end products (sushi-grade tuna, steak tartare, etc.) if that’s what the cost structure requires, before scaling and tackling more mainstream products. Thus, it is not at all clear that 100 kTA is an appropriate threshold for assessing economic viability against commodity meat products, and, as Humbird notes, “further cost reduction is likely at larger scale.”
The projected growth factor cost is high and few hydrolysate sources are considered.
The cost contribution for media in the Humbird report is higher than the CE Delft estimates, and only soy is discussed as a possible source of hydrolysates. One of the cultivated meat companies we consulted also pointed out that other plant-based protein sources, such as potato protein, could serve as suitable amino acid or hydrolysate sources with less processing. The industry will almost certainly select a combination of hydrolysates and extracts for the bulk supply of amino acids and vitamins. We need more research to understand what sources and combinations of hydrolysates make the most sense in terms of both a metabolic fit for the cell types to be used as well as supply chain, cost, and sustainability considerations. A number of noteworthy business partnerships between cultivated meat companies and major suppliers of raw materials are promising indicators that these considerations are indeed being acted upon (see, for example, the relationships that Mosa Meat, Blue Nalu, Meatable, and Aleph Farms have established with suppliers of animal feed, food ingredients, and specialty chemicals such as Nutreco, Griffith Foods, DSM, and Mitsubishi Corporation). Early data presented at the 2021 Good Food Conference by a Nutreco representative suggests that food-grade amino acids can perform just as well as pharma-grade sources, likewise for feed-grade glucose that is even more cost-effective. Further research assessing food- and feed-grade media components is warranted, but early results suggest that their inclusion in media is tractable, despite what is claimed in The Counter piece.
Similarly, the cost contributions of growth factors within the Humbird model seem unlikely to reflect the anticipated costs by the time the CM industry reaches commercial-scale production. The calculations don’t take into account the costs for proteins that are non-USP/cGMP-grade, presumably because these data aren’t readily available, but manufacturers of these proteins confirm that certifications often account for a substantial fraction of the difference in price among various recombinant proteins. A number of innovators are exploring growth factor production platforms that may offer drastic cost reductions, such as recombinant production in plants or using cell-free expression systems. Recent presentations at cultivated meat conferences suggest that plant production platforms for growth factors could result in reduced costs three orders of magnitude in a 5-year timeframe, a dramatic difference from the estimated prices cited in Humbird’s analysis. Similar results were presented at the 2021 Good Food Conference for growth factors produced via conventional microbial fermentation. We are currently working with scientists at four different growth factor manufacturers to co-author a forecasting article for growth factor cost reductions based on four different production platforms to determine how quickly and at what volumes these platforms may be able to achieve the projected price points.
Equipment costs and scaling factors disagree with other studies and do not account for industry-wide pricing changes with scale.
A 20kL bioreactor in a recent techno-economic analysis from UC Davis, Risner et al., is estimated to be $1MM USD — a 50% difference from the $1.5MM USD cited by Humbird. In the study by Risner et al., a Lang factor of 2 was chosen for all scenarios to represent a food/bioprocessing facility that could be easily configured for cultivated meat production. They note in the Supplementary discussion that this is probably low and a Lang factor of 3-5 would likely be more appropriate, which is in the range cited by two independent groups we consulted with. This still is not as high as Humbird’s Lang factor of 6.6.
Another consideration is how the cost of production of large-scale animal cell bioreactors (i.e. ≥10kL) may be affected by future demand. Back of the envelope calculations by Mark Post suggest that to supply 1% of the global demand for meat in 2030 (i.e. 3 million metric tons per year), one would expect a requirement for volumetric bioreactor capacity to be around 2×108 liters. Estimations by McKinsey suggest this number may be even higher — between 2.2×108 – 4.4×108 liters to reach just 0.5% of global meat demand — roughly 11-22x larger than the volumetric capacity of the current global pharmaceutical industry. These numbers imply that many thousands of new large-scale bioreactors will need to be manufactured to meet this demand. The mass production of this equipment would be expected to lower the unit cost for each bioreactor due to economies of scale, more efficient production, and higher demand. Assessment of other industries where large demand for stainless steel or high-grade plastic has materialized on relatively short timescales may provide better insight into cost projections for future bioreactors than examining current costs at low production volumes. There is also the possibility for technological advances in manufacturing technologies for these infrastructure components that could substantially affect costs (George Peppou of Vow gives the example of SpaceX’s pioneering of novel stainless steel welding techniques.) Furthermore, the current production costs and volumes of stainless steel bioreactors may also be affected by recent double-digit growth of the single-use bioreactor industry, which may have disincentivized upscaled manufacturing capacity for stainless steel bioreactors over the past decade.
Only growth of adult stem cells in single-cell suspension is considered.
There is no discussion of aggregate suspension culture, and the notion of using porous microcarriers that could be integrated into the final product rather than necessitating cell detachment is fairly summarily dismissed. Growth of cells on microcarriers or within other encapsulated environments could be essential to scale-up, as some data suggests that shear stress forces experienced in ultra large scale bubble column and air-lift reactors may be able to be tolerated when cells are grown on microcarriers (Li, 2020). These assumptions might make sense in typical biomedical cell culture processes, but they are being actively challenged by multiple contenders in the cultivated meat field. For example, cells can proliferate to high density within porous or degradable scaffolds, which could be used in the form of microcarriers, encapsulated tubes or spheres, or incorporated into perfusable bioreactors. As long as the scaffolds are edible, they could be used for both proliferation and maturation stages, overcoming many of the challenges regarding harvesting efficiency and other aspects of transition between these two phases. While there is still much R&D to be done, limiting the analysis to single-cell suspension systems is not reflective of the current approaches within cultivated meat companies. Additionally, it is assumed that the cells used will be immortalized adult stem cells, whereas companies are in fact pursuing a diverse set of approaches, including the use of ESCs and iPSCs. These additional approaches offer their own advantages and challenges in terms of metabolism and appropriate growth conditions.
There is insufficient discussion of media recycling or waste valorization prospects.
Density criticisms using stoichiometry are influenced by the rate of ammonia (and less so lactate) production. A discussion on how/whether the industry may capture these metabolites and perhaps even offset costs with them is probably warranted. Modeling a scenario where ammonia is captured at the rate it is produced, or at least where its production is slowed, would be useful. In our discussions, many companies do intend to implement some form of media recycling or metabolic shunting and there is active research ongoing in the area by several companies, so greater details should be gathered from cultivated meat companies on the prospects for media recycling rather than dismissing it as unachievable.
A reduction in ammonia or lactate concentrations is not limited to externally-applied recycling systems either. A patent from Memphis Meats, for instance, describes the engineered overexpression of glutamine synthetase within cells to reduce ammonia concentrations by up to 20% while increasing the supply of the important amino acid glutamine as a byproduct of the enzymatic reaction. Other animal cells, such as those from avian species, are naturally more tolerant to ammonia concentrations due to higher expression levels of the same glutamine synthetase enzyme (Stern and Mozdziak, 2019). Scenario analyses around overcoming assumed stoichiometric limitations are thus warranted.
Approaches to improve oxygen carrying capacity are not discussed.
In animal bodies, cells of course grow incredibly densely within tissues. This is only possible because of vasculature and, importantly, the presence of oxygen-carrying molecules in blood. There is precedent for using oxygen carriers in animal cell culture to increase oxygen transfer rates and thus OUR and cell density, but this is not mentioned in this analysis. Oxygen is often the rate-limiting substrate within a bioreactor, as approximately 45 times the amount of dissolved oxygen can be carried by blood versus cell culture media (Martin and Vermette 2005). For cultivated meat, the addition of recombinant heme proteins (Zhao, 2020) would be attractive not just for their oxygen carrying capacity but also their contribution to flavor, color, and cooking profiles via the Maillard reaction (Simsa et al, 2019).
Computational methods may drastically accelerate solutions for scale-up.
Robust computational models can accelerate the time to finding solutions and avoiding incorrect paths during scale-up. These methods were not available, at least at sufficient quality, until very recently, so it seems inappropriate to assume that optimization to suit the needs of this emerging industry will require the same time and resources as for other new industries in the past. Serious efforts are already underway to leverage computational methods for the acceleration of cultivated meat research. For example, the Cultivated Meat Modeling Consortium aims to address many scale-up challenges (Li, 2020), including bioreactor design, sensor equipment, feeding strategies, and medium formulations using computational methods to reduce the need for expensive, time-intensive empirical experimentation.
Despite decades of optimization, CHO cells don’t represent the limits of possibility with regard to cell line development for cultivated meat.
This analysis frequently references data from CHO cells as a benchmark for understanding the limits of cell line optimization: because CHO cells have been thoroughly characterized and optimized for over 60 years, the insinuation is made that further optimization will produce only incremental improvements in production efficiency. However, this assumption only makes sense if the relevant metrics for the cultivated meat industry are the same as those for which CHO production has been optimized in the past. In the pharmaceutical industry, CHO cell biomass is essentially a waste product. Cell line characteristics and culture methods have been optimized to increase the desired secreted product while minimizing CHO cell biomass, since any metabolism directed toward cell biomass production is considered undesirable. For production of cultivated meat, the goal is to increase biomass while reducing the production of secreted proteins and metabolites. Optimization of cell lines for this purpose will therefore have opposite goals compared to what has been done with CHO cells in the past. This limitation is acknowledged briefly, yet the paper continues to draw comparisons to CHO cells and facilities, which should be made with extreme care.
Authors
Liz Specht, Ph.D.
DIRECTOR OF SCIENCE AND TECHNOLOGY
Liz Specht oversees GFI’s Science and Technology department to build a roadmap for accelerating alternative protein research while empowering scientists to execute on this vision.
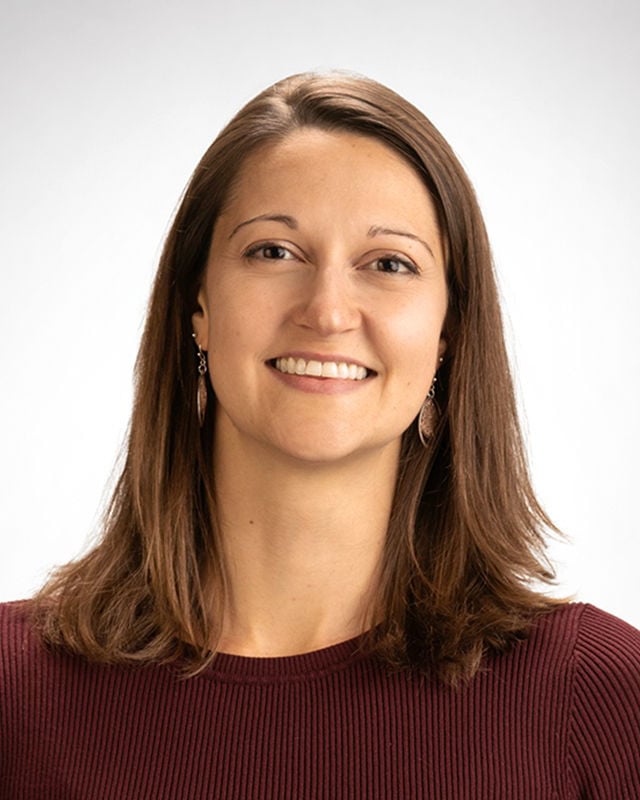
Elliot Swartz, Ph.D.
LEAD SCIENTIST, CULTIVATED MEAT
Elliot Swartz analyzes scientific progress and bottlenecks in cultivated meat.
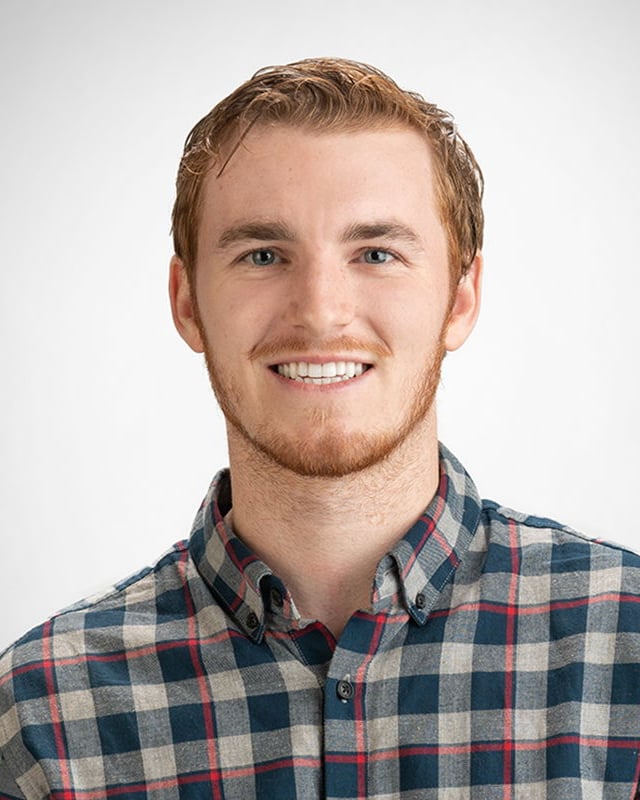
Claire Bomkamp, Ph.D.
SENIOR SCIENTIST, CULTIVATED SEAFOOD
Claire Bomkamp is focused on cultivated seafood and driving forward GFI’s Sustainable Seafood Initiative.
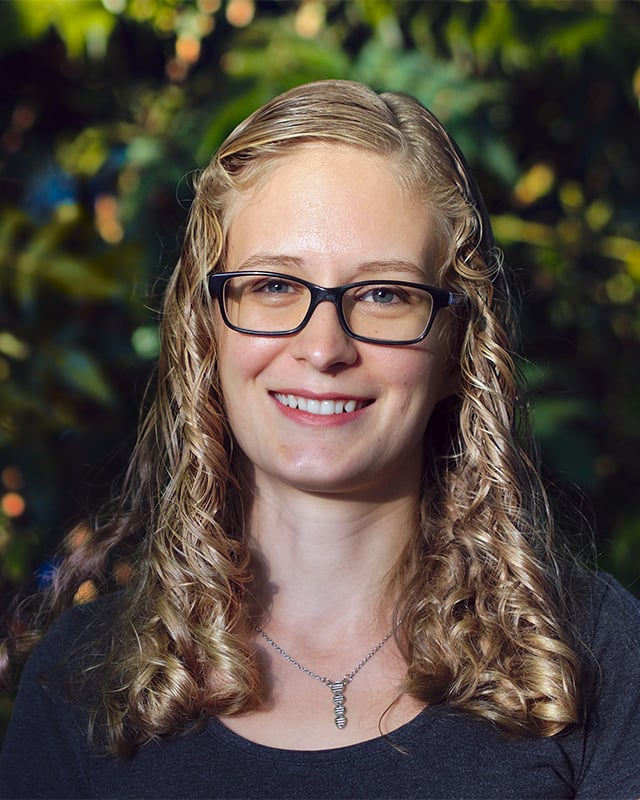
Header image courtesy of Upside Foods