Scaffolds and structural approaches to optimize fat distribution and content in cultivated meat
The inclusion of fat and marbling in cultivated meat is likely to increase its flavor, texture, and consumer appeal. Structural approaches using edible microcarriers, hydrogels, and 3D bioprinting present promising options to support fat cell growth and reduce buoyancy in culture for integrating fat into cuts of meat, but more research is needed to optimize conditions.
-
Cultivated
- Research
- Commercial
- End Products
- Production
- R&D
- Raw Materials, Ingredients, & Inputs
- Bioprocess design
- End product formulation & manufacturing
- Scaffolding
- Industry
- Academics
- Startups
Current challenge
Incorporating fat and muscle cells into whole muscle cuts like steaks will be vital for cultivated meat’s taste and, ultimately, increasing consumer adoption. Fat is prized in structured cuts of meat and contributes to high-end rating systems such as the one used for Wagyu beef, which ranks fat distribution and marbling qualities. The extent of marbling in conventional meat, or the amount of intramuscular fat embedded in muscle tissue, depends on several factors such as animal genetics, environment, and cut of meat. Fat has desirable organoleptic properties and nutrients but conventional animal fat can pose risks associated with bioaccumulation of toxins and pollutants. Plant-based fat provides flavor but has different chemical properties than animal fat like lower melting points, which change its cooking qualities. Cultivated fat could provide the same cooking characteristics, organoleptic properties, and nutritional value as conventional fat, while reducing the risk of harmful animal-based contaminants. Integrating fat into structured cuts of cultivated meat is a complex process. One approach is to co-culture myocytes and adipocytes onto a structured scaffold. As with scaffolds for muscle, scaffolds for fat need to be cost-effective, maintain organoleptic properties, and—if edible and intended to remain integrated in the end product— to assist with taste, texture, and nutrition. Muscle and fat cells, however, require different conditions to grow and differentiate, including different cell media formulations and mechanical environments. When co-cultured together, fat cells may suppress muscle cell differentiation and could cause proinflammatory cytokine expression in muscle cells, though some reports contradict these findings. Adipogenic cells pose a unique challenge compared to myocytes because mature fat cells become buoyant and anchorage-dependent after differentiation. Differences in these physical properties indicate that different topographic cues—such as scaffold stiffness or adhesive properties—may be needed for muscle and fat cell differentiation. The scalability of creating marbled cultivated meat is also untested.
Proposed solution
There are several approaches for fat integration into cultivated meat. Generally, fat is either prepared separately from other components and then combined together for a finished product (e.g., Mission Barns, Hoxton Farms, Cubiq Foods) or prepared as one 3D co-cultured structure (e.g., Meatable). Additional research is needed to determine optimal co-culture conditions for adipose and myoblast cells. Adipose cells grown in 3D culture display qualities more similar to in vivo tissue than when grown in 2D culture, so 3D scaffolds will likely be needed for optimal fat formation. There are a variety of structural approaches and scaffold materials that could facilitate the production of marbled cultivated meat, but additional research is needed in all of these domains to determine the most promising paths.
Microcarriers in suspension bioreactors can induce spheroid formation, enabling adipose adherence that facilitates cell proliferation without a bulky scaffold. While microcarriers are inexpensive and can be made of edible materials, this technology has limited scalability and requires extended incubation periods. Free-floating microcarriers for adipose cells may also require a specialized bioreactor (such as spinning flask) for constant stirring or must be weighted to reduce fat cell buoyancy. Growing cultivated fat as small aggregates that are later combined into a final product side-steps the need to mimic the function of the vasculature in larger 3D cultures because oxygen, nutrient, and waste transport can be achieved by diffusion. Smaller cultivated fat pieces could also be ideal for blended cultivated fat/plant-based products like sausage, meatballs, and nuggets, as fat plays an important role in giving meat its flavor and juiciness.
Hydrogels offer a structured integration system and a variety of scaffold material options. Thermoreversible 3D hydrogels may provide a scalable approach, addressing the mature adipose buoyancy problem by constraining cells within the matrix. Flexible hydrogels increase lipid accumulation and differentiation, but stiff materials promote adipose progenitor cell self-renewal. Synthetic polymers are inexpensive and versatile but require removal before consumption, which can cause significant volume and cell loss. Alginate derived from algae is an edible, flexible polymer with promising organoleptic properties. Chitosan (from crustacean shells or fungi) and native extracellular matrices (animal and plant-derived) are hydrogel materials, but animal-derived extracellular matrices like fibrin and collagen would need to be substituted by recombinant versions for use as cultivated meat scaffolds. Varied mechanical characteristics like modulated material stiffness with flexible regions that support adipogenesis, different cross-linking ratios, and varying the molecular weight of the material can alter scaffold conditions and enable fat marbling.
Porous scaffolds like freeze-dried bacterial nanocellulose provide animal-free 3D macroporous structures that are compatible with adipocytes. The porous qualities of apples coated with poly-L-lysine also enable adipose cell adhesion. Applying various growth factors in co-cultures could trigger cell differentiation in different scaffold regions and help control fat distribution in cultivated meat.
Bioprinting is a promising approach for fat distribution in cultivated meat—whether as a method for spatially distributing cells and scaffold biomaterials or the patterning of differentiated cells into a final product—that companies like Peace of Meat (owned by MeaTech) and Ants Innovate are implementing. Ants Innovate describes proprietary tissue building blocks or “cartridges” used to assemble muscle and fat into meat cuts. The first cultivated steak reported in academic literature was created with a novel tendon-gel integrated bioprinting method that included muscle, fat, and vascular tissue. The different tissue types were not co-cultured but physically assembled by stacking and cross-linking the tissue fibers. The authors hypothesized, however, that if all of the cell types could be co-cultured, then bioprinting could be used to dispense co-cultures into patterned fat marbling. Plant-based biomaterials are being explored as nutritious, compatible scaffolds to facilitate bioprinting. Some efforts are underway to combine different scaffold types in order to optimize structure and vascularization. For example, printed electrospun fibers can press texture onto beads to create microgrooved microbead scaffolds for myocytes and can be combined with self-assembling edible hydrogel microbeads containing adipocytes.
Table of scaffold types and materials for adipocytes.
Scaffold types for fat
Source | Examples | Advantage | Disadvantage | |
---|---|---|---|---|
Microcarriers | Variety | Muoio et al., 2021; Ashok et al., 2016 | Inexpensive, minimal additives, facilitate self-assembling spheroids | Long incubation, low scaling potential, must be removed if inedible material |
Hydrogel | Variety | Contessi Negrini et al., 2019; Kim et al., 2010; Mehta et al., 2019 | Structured integration | Additional processing required to produce heterogeneous constructs |
Porous scaffolds | Variety | Contessi Negrini et al., 2020; Krontrias et al., 2015 | Animal-free, strong vascularization | Chemical processing for functionalization |
Scaffold materials for fat | ||||
Synthetic polymers | Chemical synthesis | Patrick et al., 1999 | Inexpensive, consistent, customizable | Inedible, could affect organoleptic properties, must be removed |
Native ECM | Animal sources or recombinant | Hume et al., 2018 | Edible, natural source for cell growth | Expensive, some animal-derived,variability |
Alginate | Brown seaweed | Contessi Negrini et al., 2019; Kim et al., 2010; Mehta et al., 2019 | Edible, inexpensive, flexible, improve organoleptic properties | Cell adhesion challenge, chemical processing for functionalization |
Chitosan | Crustaceans, fungal mycelia | Wu et al., 2007 | Edible, common food additive, antimicrobial properties, animal-free if fungal-derived | Chemical conversion to chitin, variability |
Bomkamp, C., Skaalure, S. C., Fernando, G. F., Ben‐Arye, T., Swartz, E. W., & Specht, E. A. (2022). Scaffolding biomaterials for 3D cultivated meat: Prospects and challenges. Advanced Science, 9(3), 2102908. https://onlinelibrary.wiley.com/doi/full/10.1002/advs.202102908
Fish, K. D., Rubio, N. R., Stout, A. J., Yuen, J. S., & Kaplan, D. L. (2020). Prospects and challenges for cell-cultured fat as a novel food ingredient. Trends in food science & technology, 98, 53-67. https://www.ncbi.nlm.nih.gov/pmc/articles/PMC7051019/
Scaffold materials for fat
Source | Examples | Advantage | Disadvantage | |
---|---|---|---|---|
Synthetic polymers | Chemical synthesis | Patrick et al., 1999 | Inexpensive, consistent, customizable | Inedible, could affect organoleptic properties, must be removed |
Native ECM | Animal sources or recombinant | Hume et al., 2018 | Edible, natural source for cell growth | Expensive, some animal-derived,variability |
Alginate | Brown seaweed | Contessi Negrini et al., 2019; Kim et al., 2010; Mehta et al., 2019 | Edible, inexpensive, flexible, improve organoleptic properties | Cell adhesion challenge, chemical processing for functionalization |
Chitosan | Crustaceans, fungal mycelia | Wu et al., 2007 | Edible, common food additive, antimicrobial properties, animal-free if fungal-derived | Chemical conversion to chitin, variability |
Bomkamp, C., Skaalure, S. C., Fernando, G. F., Ben‐Arye, T., Swartz, E. W., & Specht, E. A. (2022). Scaffolding biomaterials for 3D cultivated meat: Prospects and challenges. Advanced Science, 9(3), 2102908. https://onlinelibrary.wiley.com/doi/full/10.1002/advs.202102908
Fish, K. D., Rubio, N. R., Stout, A. J., Yuen, J. S., & Kaplan, D. L. (2020). Prospects and challenges for cell-cultured fat as a novel food ingredient. Trends in food science & technology, 98, 53-67. https://www.ncbi.nlm.nih.gov/pmc/articles/PMC7051019/
Methods for fat distribution
Source | Examples | Advantage | Disadvantage | |
---|---|---|---|---|
Co-culturing | Various myocytes, adipocytes, endothelial cells | Ben-Arye et al., 2020; Choi et al., 2013; Yan et al., 2013; Cui et al., 2018 Kuppusamy et al., 2021 | Allows natural cell signaling to occur during development, no end-stage combination required | Different conditions needed for different cell types |
Bioprinting | Variety | Kang et al., 2020 | Precise distribution | Machine and programming dependent |
Growth factor supplementation | Variety | Banks et al., 2014; Dinis et al., 2014; Mvula and Abrahamse, 2014 | Directed differentiation | Expensive additive |
Tuning mechanical stiffness | Variety | Chandler et al., 2011; Freeman and Kelly, 2017; Banks et al., 2014 | No additives | Materials must be compatible, may produce mixtures of cell types |
Bomkamp, C., Skaalure, S. C., Fernando, G. F., Ben‐Arye, T., Swartz, E. W., & Specht, E. A. (2022). Scaffolding biomaterials for 3D cultivated meat: Prospects and challenges. Advanced Science, 9(3), 2102908. https://onlinelibrary.wiley.com/doi/full/10.1002/advs.202102908
Fish, K. D., Rubio, N. R., Stout, A. J., Yuen, J. S., & Kaplan, D. L. (2020). Prospects and challenges for cell-cultured fat as a novel food ingredient. Trends in food science & technology, 98, 53-67. https://www.ncbi.nlm.nih.gov/pmc/articles/PMC7051019/
Anticipated impact
Producing marbled cultivated meat is expected to improve the flavor and texture of the final product while increasing consumer acceptance. Advances in cultivated fat incorporation would enable more variety and structural complexity of cultivated meat products and could facilitate the creation of familiar cuts of meat. For example, if bioprinting becomes a primary method of integrating fat into cultivated meat, producing marbled cultivated meat could depend on printer programming capabilities and access to bioprinting devices. Marbled meat could become restricted by the total number of bioprinters, but with recent scaling in 3D printing, bioprinters could become readily available, easy to use, and portable. Certain scaffold types and materials may carry advantages for specific forms of marbled cultivated meat, so different combinations of scaffolds may be warranted. Additional research is needed to optimize scaffold conditions for fat. Cultivated fat could reduce foodborne illnesses, contaminants, trans fats, and antibiotic use while providing beneficial organoleptic qualities to cultivated meat.
Related efforts
Engineered whole cut meat-like tissue by the assembly of cell fibers using tendon-gel integrated bioprinting. (Kang et al., 2021)
Moo’s Law: An Investor’s Guide to the New Agrarian Revolution. (Jim Mellon, 2020)
There is precedent for complex co-culture approaches involving multiple cell types. For example, a triculture with satellite, smooth muscle, and endothelial cells demonstrated myogenesis and scaffold adherence.
Relevant review articles:
- Scaffolding biomaterials for 3D cultivated meat: Prospects and challenges. (Bomkamp et al., 2022)
- Prospects and challenges for cell-cultured fat as a novel food ingredient. (Fish et al., 2020)
- Perspectives on scaling production of adipose tissue for food applications. (Yuen et al., 2022)
Additional resources:
GFI resources
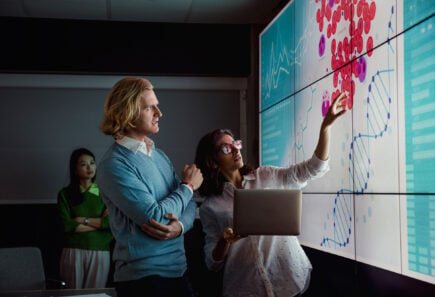
Collaborative Researcher Directory
Use this directory to find scientific collaborators in the alternative protein field.
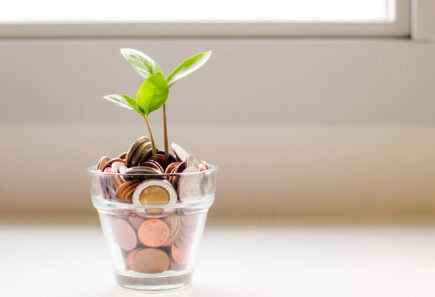
Research funding database
GFI’s research funding database provides curated grant opportunities for open-access alternative protein research.
Meet the author
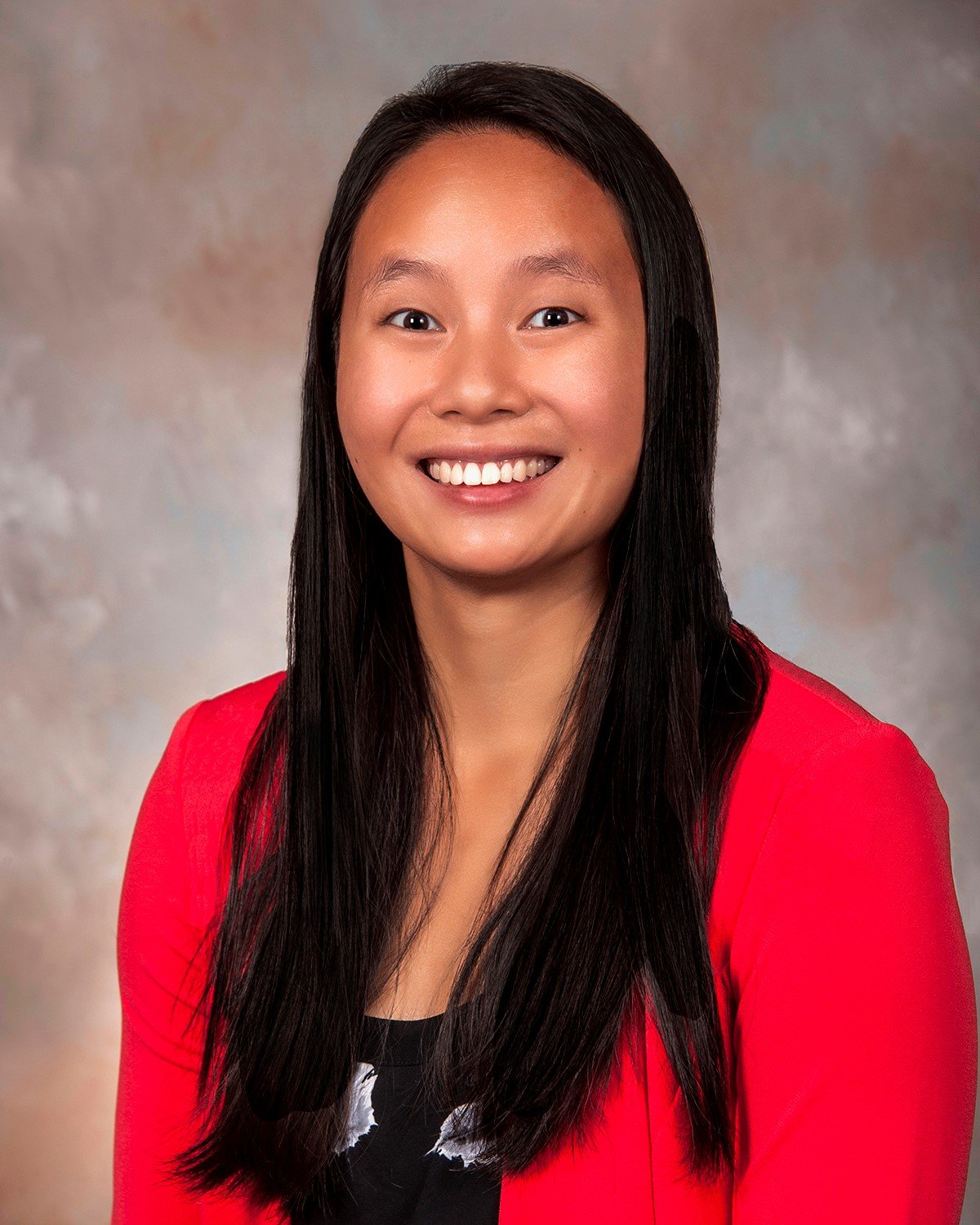
Eileen McNamara
GFI RESEARCH FELLOW
Uniformed Services University of the Health Sciences
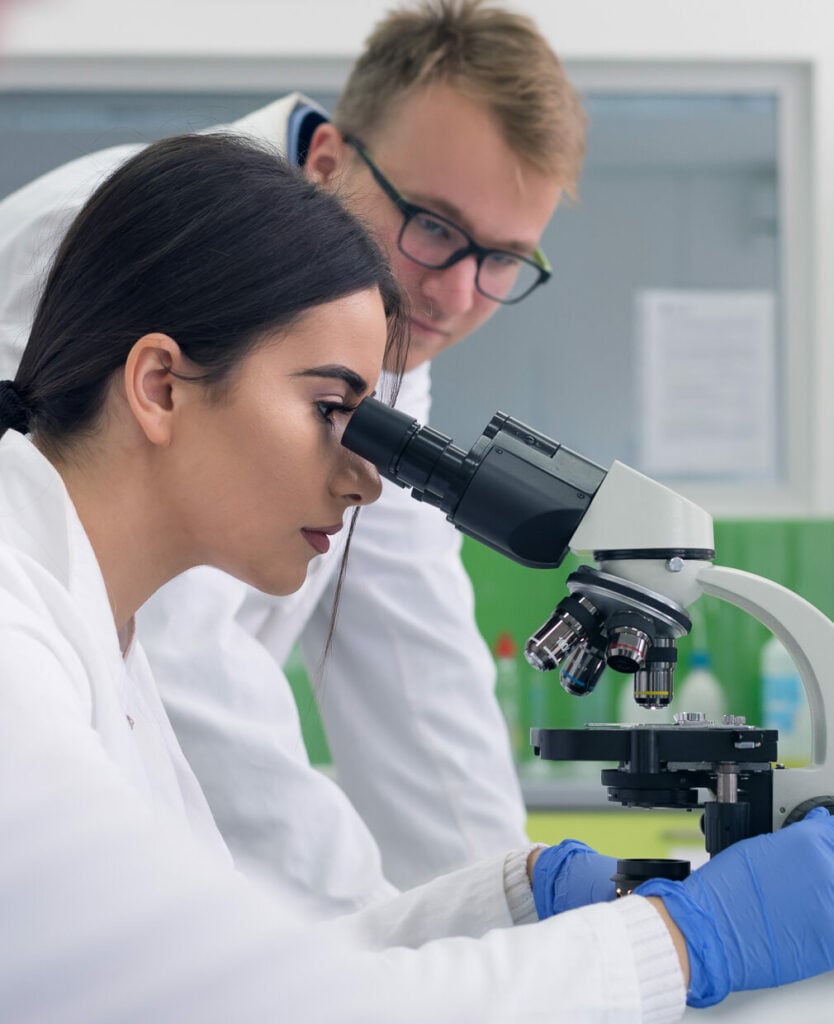
Find collaborators
Join the GFIdeas global community of 2,000+ entrepreneurs, scientists, investors, and subject matter experts. Discuss projects on the members-only Slack community, attend monthly seminars, and use the community directory to help you find collaborators working on similar Solutions!
Related solutions
-
Cultivated
Incorporating growth factors into scaffolds to reduce costs and introduce spatial heterogeneity
Growth factors (GFs) can be incorporated into scaffolds as a strategy for both reducing costs and improving product quality of cultivated meat. Open-access research is needed to test the feasibility…
-
Cultivated
-
Fermentation
-
Plant-Based
Optimizing fat profiles for nutritional and sensory properties
Because alternative meat’s fat content and fatty acid profile can be more easily controlled than conventional meat’s, there is an opportunity to alter fat content for nutritional benefits. Additional research…
Related GFI research grants
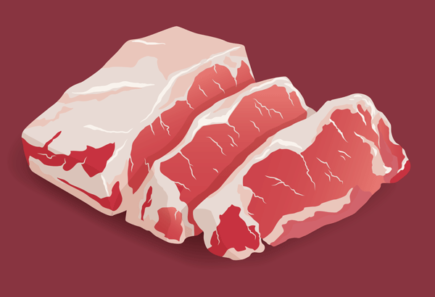
Developing marbled cultivated beef
GFI is developing marbled cultivated beef with Dr. Amy Rowat at University of California, Los Angeles
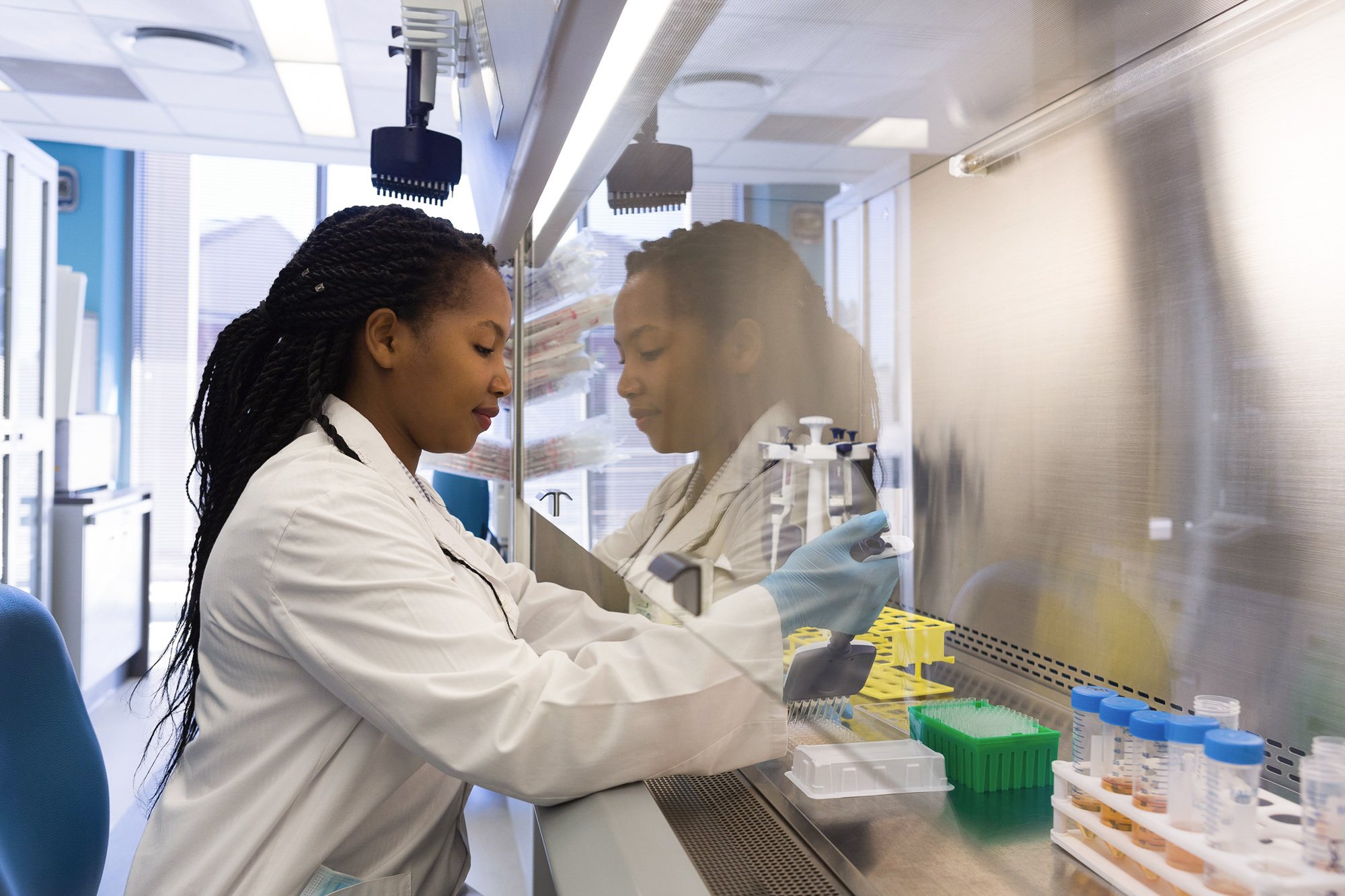
Explore the full solutions database
Browse 100+ startup ideas, commercial opportunities, research projects, and investment priorities throughout the alternative protein supply chain.
Get involved
If you’d like to fund a research project, work on any of these solutions, share information about related efforts that are already underway, or elevate new ideas for advancing the alternative protein industry, we’d love to hear from you!