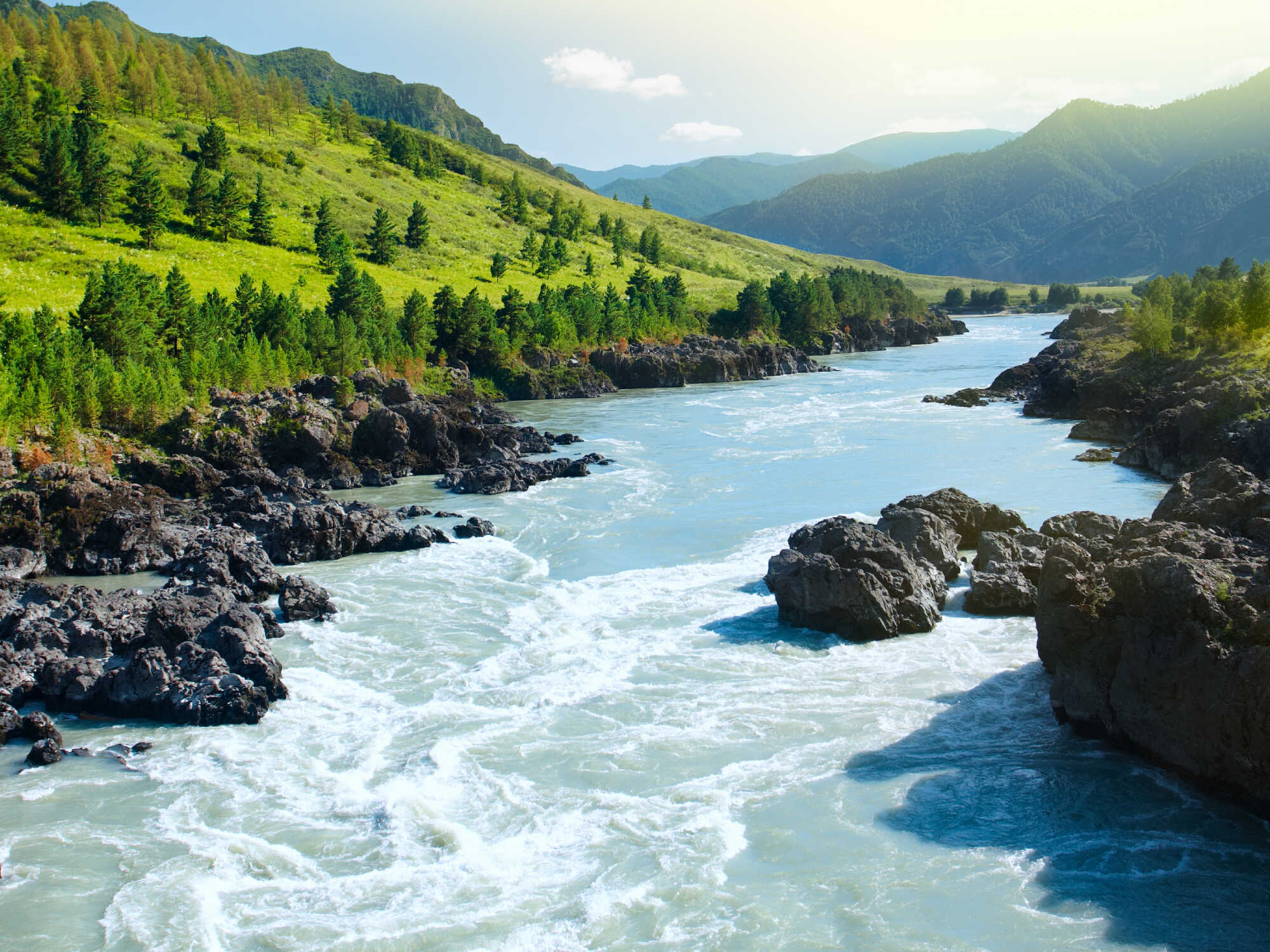
Environmental impacts of alternative proteins
Alternative proteins can reduce the environmental impact of our food system by conserving land and water and minimizing greenhouse gas emissions and other pollution. With sufficient public investment, alternative proteins will help deliver a sustainable and secure food supply.
Save this as a PDF
Diversifying the global food supply with alternative proteins will safeguard our natural resources
Global demand for meat is expected to rise by at least 50 percent by 2050.1 Meeting this demand with conventional meat alone would accelerate pollution and deplete scarce natural resources. Alternative proteins—meat and seafood made from plants, fermentation, or cultivated from animal cells—use a fraction of the land and water required by conventional meat, generate fewer greenhouse gases (GHGs), and reduce the flow of pollutants into communities and ecosystems. To safeguard our natural resources, policymakers should support alternative proteins by making public investments in research, development, and commercialization.
Comparative life cycle assessments
Explore the profound environmental benefits of alternative proteins through insights derived from our in-depth analysis of Life Cycle Assessment (LCA) studies. Delve into the accompanying table to gain a nuanced understanding of the distinct sustainability advantages associated with various alternative protein products.
This table presents the results of publicly available, externally commissioned industry Life Cycle Assessment (LCA) studies published to date, as well as relevant peer-reviewed academic LCAs published January 2021–August 2023, that compare alternative protein products with conventional meat products. Due to differences in methodology, the results from different studies cannot be precisely compared. Impact reductions are calculated as follows: (impact of conventional meat product ﹣ impact of alternative protein product) ÷ (impact of conventional meat product). Negative (-) values indicate reductions in impact within the environmental category. Positive (+) values indicate increases in impact. A dash (—) sign indicates data is unavailable. * Raw/uncooked product. ** Cooked product. † Includes the carbon opportunity of reduced land use. All other studies do not calculate the carbon opportunity of reduced land use. If freed-up land is used to mitigate climate change, benefits will be significantly greater than the direct impact reductions measured. Δ For Sinke, et al. (2023), the 2030 scenarios are based on conservative (higher resource use) assumptions of future cultivated meat commercial-scale production coupled with ambitious, low carbon footprint benchmarks for conventional meat production. The cultivated meat products evaluated were produced with renewable energy.
Alternative proteins are land-efficient
Alternative proteins use resources directly, without cycling them through animals, and thus require significantly less land. Plants provide nearly two-thirds of the global protein supply on only one-fourth of all agricultural land.2 A shift to alternative proteins could free up twice as much land as China and India combined.3 With reforestation and carbon sequestration, the freed-up land would further mitigate climate change—potentially removing 26 gigatons of carbon dioxide equivalent per year, about half of current global emissions.4 Moreover, by mitigating deforestation and habitat loss, alternative proteins can serve as a key strategy to preserve biodiversity.
Alternative proteins generate fewer GHGs
Alternative proteins have significantly smaller GHG footprints as they do not require raising methane-emitting livestock and growing crops for feed. They provide a crucial tool to decarbonize food systems—which account for over a third of global GHG emissions—and meet Paris Agreement goals.5 If alternative proteins rise to half the global protein market, including dairy, they would mitigate 5 gigatons of carbon dioxide equivalent annually, and agriculture and land-use GHG emissions would decline by 31 percent by 2050 instead of increasing.6 By capturing just 11 percent of the protein market by 2035, alternative proteins can slash GHGs on a scale roughly equal to decarbonizing the entire aviation industry.7
Alternative proteins are water-efficient and can address water scarcity
Only 0.003 percent of Earth’s water is available freshwater, and over two billion people currently live in countries with inadequate water supply.8 Water scarcity is projected to worsen in the coming decades, especially in drought-susceptible areas like the American West, including the Colorado River—for which more than three-quarters of water withdrawals are related to agriculture.9 In fact, agriculture is the largest user of freshwater, accounting for 70 percent of withdrawals globally.10 Plant-based meat can reduce water use by up to 99 percent and cultivated meat by 66 percent (compared to conventional beef).11 As water becomes a limited resource, alternative proteins offer a water scarcity solution.12
Alternative proteins cause significantly less air and water pollution
By using plants, fermentation, or cell cultivation instead of livestock, the production of alternative proteins does not emit the same toxic air pollutants—including ammonia, particulate matter, and hydrogen sulfide—as conventional meat production.13 Similarly, because there is no animal waste to discharge and fewer crops and fertilizers are needed, alternative proteins reduce the discharge of nitrogen and phosphorus, which stimulate the growth of algal blooms that impair water quality.14 A shift toward alternative proteins can keep our air and water clean, improving the health of communities and ecosystems while meeting increasing protein demand.
Policymakers should invest in alternative proteins for a secure, sustainable food supply
Supporting alternative protein innovation and commercialization will increase food security. Beyond their significant environmental benefits, alternative proteins expand consumer choice and promote national security by increasing supply chain resilience.15 In addition, transitioning to alternative proteins could support 83 million jobs and generate $700 billion in economic value by 2050 globally.16 Like renewable energy, technologies enabling alternative proteins are key environmental solutions made possible by scientific breakthroughs. To unlock alternative proteins’ full potential, the world’s governments should collectively invest $10.1 billion annually in research, development, and commercialization.17 With sufficient public investment, alternative proteins can deliver a secure food supply for a growing population while protecting the environment and global stability.
Addressing environmental challenges with alternative proteins
The comprehensive impact of embracing alternative proteins extends beyond addressing the above environmental issues—it is a holistic approach towards a sustainable and resilient future.
Climate
Scaling up alternative proteins is a high-impact solution to the global climate crisis.18 Animal agriculture produces 20 percent of GHG emissions globally, which come from animal feed production, animal digestion, and waste.19 Livestock alone account for nearly 40 percent of U.S. methane emissions.20 The Paris Agreement’s 1.5°C warming limitation goal cannot be met unless conventional meat consumption declines.21
Air pollution
Alternative proteins can help improve community health as they generate significantly less pollution. In the United States, a shift to alternative proteins could prevent approximately 12,000 deaths per year from a reduction in air pollution alone.22 As air pollution disproportionately impacts low-income and minority communities, alternative proteins can promote environmental justice within the food system.
Deforestation
We are running out of room for conventional protein production, which has led to the clearing of forests and other ecosystems for pasture and cropland. Shifting just 20 percent of per-capita ruminant meat consumption to alternative proteins by 2050 would offset future increases in global pasture area and cut annual deforestation—and related carbon emissions—in half.23
Biodiversity
Conversion of land to agriculture is linked to 70 percent of all projected terrestrial biodiversity loss in coming decades.24 Large-scale biodiversity loss can destabilize the water cycle, climate, and other global systems and lead to food scarcity, nutritional deficits, and economic insecurity. The land efficiency of alternative proteins can help reverse deforestation and habitat loss trends, preserving biodiversity.
References
1. Our World in Data. n.d. “Global Meat Consumption, World, 2000 to 2050.” Accessed July 19, 2023. https://ourworldindata.org/grapher/global-meat-projections-to-2050?time=2000.latest; Food and Agriculture Organization of the United Nations (FAO). 2018. “The Future of Food and Agriculture—Alternative Pathways to 2050. Supplementary Material.” Rome, Italy. https://www.fao.org/3/CA1564EN/CA1564EN.pdf.
2. Ritchie, Hannah. 2019. “Half of the World’s Habitable Land Is Used for Agriculture.” Our World in Data. https://ourworldindata.org/global-land-for-agriculture.
3. Ritchie, Hannah. 2021. “If the World Adopted a Plant-based Diet We Would Reduce Global Agricultural Land Use from 4 to 1 Billion Hectares.” Our World in Data. https://ourworldindata.org/land-use-diets.
4. Hayek, Matthew N., Helen Harwatt, William J. Ripple, and Nathaniel D. Mueller. 2020. “The Carbon Opportunity Cost of Animal-Sourced Food Production on Land.” Nature Sustainability 4 (September): 21–24. https://doi.org/10.1038/s41893-020-00603-4; Ritchie, Hannah, Pablo Rosado, and Max Roser. 2020. “Greenhouse Gas Emissions.” Our World in Data. https://ourworldindata.org/grapher/global-meat-projections-to-2050?time=2000.latest.
5. Xu, Xiaoming, Prateek Sharma, Shijie Shu, Tzu-Shun Lin, Philippe Ciais, Francesco N. Tubiello, Pete Smith, Nelson Campbell, and Atul K. Jain. 2021. “Global Greenhouse Gas Emissions from Animal-based Foods are Twice Those of Plant-based Foods.” Nature Food 2 (September): 724–732. https://doi.org/10.1038/s43016-021-00358-x; Clark, Michael A., Nina G. G. Domingo, Kimberly Colgan, Sumil K. Thakrar, David Tilman, John Lynch, Inês L. Azevedo, and Jason D. Hill. 2020. “Global Food System Emissions Could Preclude Achieving the 1.5° and 2°C Climate Change Targets.” Science vol. 370, issue 6517 (April): 705–8. https://pubmed.ncbi.nlm.nih.gov/33154139.
6. ClimateWorks Foundation and the UK Foreign, Commonwealth & Development Office. 2021. “Global Innovation Needs Assessments: Protein Diversity.” https://www.climateworks.org/wp-content/uploads/2021/11/GINAs-Protein-Diversity.pdf; Kozicka, Marta, Petr Havlík, Hugo Valin, Eva Wollenberg, Andre Deppermann, David Leclère, Pekka Lauri, Rebekah Moses, Esther Boere, Stefan Frank, Chris Davis, Esther Park, and Noel Gurwick. 2023. “Feeding Climate and Biodiversity Goals with Novel Plant-Based Meat and Milk Alternatives.” Nature Communications 14 (September): 5316. https://doi.org/10.1038/s41467-023-40899-2.
7. Morach, Benjamin, Malte Clausen, Jürgen Rogg, Michael Brigl, Ulrik Schulze, Nico Dehnert, Markus Hepp, Veronique Yang, Torsten Kurth, Elfrun von Koeller, Jens Burchardt, Björn Witte, Przemek Obloj, Sedef Koktenturk, Friederike Grosse-Holz, and Olivia Stolt. 2022. “The Untapped Climate Opportunity in Alternative Proteins.” Boston Consulting Group, MA. https://www.bcg.com/publications/2022/combating-climate-crisis-with-alternative-protein.
8. Food and Agriculture Organization of the United Nations (FAO). 2017. “Water for Sustainable Food and Agriculture: A Report Produced for the G20 Presidency of Germany.” Rome, Italy. https://www.fao.org/3/i7959e/i7959e.pdf; UNICEF. n.d. “Water Scarcity.” Accessed July 20, 2023. https://www.unicef.org/wash/water-scarcity.
9. UNICEF (see note 8); Richter, Brian D., Dominique Bartok, Peter Caldwell, Kyle Frankel Davis, Peter Debaere, Arjen Y. Hoekstra, Tianshu Li et al. 2020. “Water Scarcity and Fish Imperilment Driven by Beef Production.” Nature Sustainability 3, (April): 319-328. https://doi.org/10.1038/s41893-020-0483-z.
10. FAO (see note 8).
11. Heller, Martin C. and Gregory A. Keoleian. 2018. “Beyond Meat’s Beyond Burger Life Cycle Assessment: A Detailed Comparison Between a Plant-Based and an Animal-Based Protein Source.” Center for Sustainable Systems, University of Michigan (September). https://css.umich.edu/sites/default/files/publication/CSS18-10.pdf; Sinke, Pelle, Elliot Swartz, Hermes Sanctorum, Coen van der Giesen, and Ingrid Odegard. 2023. “Ex-ante Life Cycle Assessment of Commercial-scale Cultivated Meat Production in 2030.” The International Journal of Life Cycle Assessment 28 (January): 234–254. https://doi.org/10.1007/s11367-022-02128-8.
12. Rojanasakul, Mira, Christopher Flavelle, Blacki Migliozzi, and Eli Murray. 2023. “America Is Using Up Its Groundwater Like There’s No Tomorrow.” New York Times, August 28, 2023. https://www.nytimes.com/interactive/2023/08/28/climate/groundwater-drying-climate-change.html.
13. Hribar, Carrie. 2010. “Understanding Concentrated Animal Feeding Operations and Their Impact on Communities.” National Association of Local Boards of Health, Ohio, p. 6. https://www.cdc.gov/nceh/ehs/docs/understanding_cafos_nalboh.pdf.
14. Mateo-Sagasta, Javier, Sara Marjani Zadeh, and Hugh Turral. 2017. “Water Pollution from Agriculture: A Global Review.” Food and Agriculture Organization of the United Nations (FAO), Rome, Italy, and International Water Management Institute (IWMI), Colombo, Sri Lanka, p. 15. https://www.fao.org/3/i7754e/i7754e.pdf.
15. Swanson, Zane, Caitlin Welsh, and Joseph Majkut. 2023. “Mitigating Risk and Capturing Opportunity: The Future of Alternative Proteins.” Center for Strategic and International Studies (CSIS), Washington, DC. https://csis-website-prod.s3.amazonaws.com/s3fs-public/2023-05/230511_Swanson_Alternative_Proteins.pdf?VersionId=Za76gtRSXe0eahjwFvr5hw54uHzCXuT5.
16. ClimateWorks Foundation and the Global Methane Hub. 2023. “Global Innovation Needs Assessments: Food System Methane.” https://www.climateworks.org/ginas-methane.
17. ClimateWorks Foundation and the UK Foreign, Commonwealth & Development Office. 2021. “Global Innovation Needs Assessments: Protein Diversity.” https://www.climateworks.org/wp-content/uploads/2021/11/GINAs-Protein-Diversity.pdf.
18. Morach, et al. (see note 7).
19. Xu, et al. (see note 5).
20. U.S. Environmental Protection Agency (EPA). 2020. “Inventory of U.S. Greenhouse Gas Emissions and Sinks: 1990-2018.” Washington, DC. EPA 430-R-20-002. https://www.epa.gov/sites/default/files/2020-04/documents/us-ghg-inventory-2020-main-text.pdf.
21. Clark, et al. (see note 5).
22. Domingo, Nina G. G., Srinidhi Balasubramanian, Sumil K. Thakrar, Michael A. Clark, Peter J. Adams, Julian D. Marshall, Nicholas Z. Muller et al. 2021. “Air Quality–related Health Damages of Food.” Proceedings of the National Academy of Sciences 118, no. 20: e2013637118. https://doi.org/10.1073/pnas.2013637118.
23. Humpenöder, Florian, Benjamin Leon Bodirsky, Isabelle Weindl, Hermann Lotze-Campen, Tomas Linder and Alexander Popp. 2022. “Projected Environmental Benefits of Replacing Beef with Microbial Protein.” Nature 605 (May): 90–96. https://doi.org/10.1038/s41586-022-04629-w.24. Almond, Rosamunde, Monique Grooten, and Tanya Petersen (Eds.). 2020. “Living Planet Report 2020.” WWF, Switzerland, p. 61. https://files.worldwildlife.org/wwfcmsprod/files/Publication/file/279c656a32_ENGLISH_FULL.pdf?_ga=2.225298906.1368214743.1689623561-1909943766.1687975692; Secretariat of the Convention on Biological Diversity. 2014. “Global Biodiversity Outlook 4.” Montréal, p. 10. https://www.cbd.int/gbo/gbo4/publication/gbo4-en-hr.pdf.